by Astrogator Mike | Dec 5, 2013 | General Comments
Our Chinese colleagues launched a lander to the Moon on December first, but unfortunately they have chosen not to publicly share where their spacecraft is. A few days ago there were several TLEs in Spacetrack associated with the Chang’e 3 launch, all of which didn’t appear to be associated with it at first glance (wrong plane, etc.) . So, given we don’t have any real ephemeris available, let’s see if we can create a reasonable guess on our own with a bit of Astrodynamics detective work.
First, let’s start off with some information we do know from their live broadcast.
We know the launch time: 1 Dec 2013 17:30 UTC.
We know the launch site is the LC2 Launch Complex at the Xichang Satellite Launch Center (XSLC). I have 28.2455 deg N Latitude, and 102.027 E Longitude for that site.
Next question is, what direction do they launch, and to what altitude?
Robert Christy’s excellent site Zarya.info provides us with a decent first guess here:
Chang’e 3 Launch Events
Robert did a lot of our work for us by nailing the separation events, and approximate latitude and longitude positions of events based on the video stream. It may seem rough to try to calculate a trajectory from this information, but actually it tells us quite a bit. We know the launch site, and we know the approximate perigee altitude of the transfer trajectory (~210 km) . We also have a pretty good idea of the TLI time: 6 Dec 2013 09:30 UTC from a tweet by Robert Christie (@Zarya_Info) and we know the landing site from the same source. So let’s make a few simplifications:
Impulsive maneuvers. I’m going to use these for TLI and LOI for now, just because I’m lazy and don’t feel like digging out the parameters of the spacecraft (and it makes my setup a bit more complicated).
From Robert’s link about, I can eyeball the center of TLI at about 17:45:36 UTC on Dec 1, 2013. That’s where I’m going to put my TLI. I don’t know the exact launch azimuth they flew, so I’m going to guess 97.5 and then let it float a bit (i.e. use the burnout Lat, Lon and Alt as controls). I don’t know their exact burnout altitude, so I’m going to guess 210. Normally I’d know all of this stuff exactly. If I were planning the mission (as I did on LADEE) I knew my launch site, azimuth etc. and the exact burnout state of the Mintoaur V. I could figure out from this what my launch time ought to be, and when TLI was etc. In this case, I’m having to guess things from parameters I DO know. It’s detective work, but my peers in China have to work with the same physics and math as me, so it’s going to be pretty close.
So I have to fix the launch time and the TLI time, let the burnout conditions float a bit for my controls, and then vary my TLI Delta-V to hit an LOI at the proper time and get into the proper orbit (I’m going to use a 100 km altitude, 90 deg inclination orbit).
So what does that give us?
First off, it makes our launch ground track look like this:
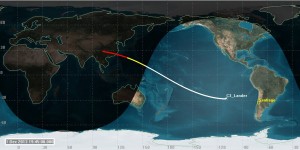
Estimated Chang’e 3 Ground track (click to zoom)
TLI occurs at the end of the yellow segment, burnout of stage 2 at the end of the red segment.
So assuming a 90 deg inclination, 100 km altitude insertion (impulsive still) on 6 Dec 2013 9:30 UTC, we get a trajectory that looks like this:
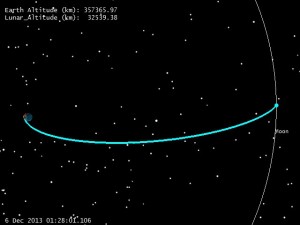
Earth-Centered View, C-3 at 5 Dec 2013 17:32 UTC (Click to zoom)
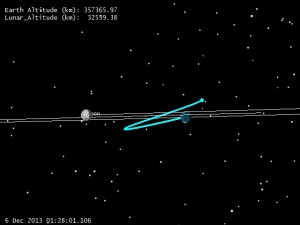
Earth-Centered View, C-3 at 5 Dec 2013 17:32 UTC (Click to zoom)
This gives us a good idea where C-3 is now, but what does the rest of the trajectory look like? First let’s look at the LOI geometry.
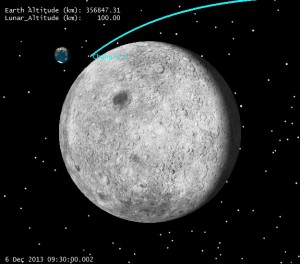
Chang’e 3 LOI Geometry (Click to zoom)
Note that with a 5 day transfer(4.8 really, 116 hrs) the approach to the Moon comes from the side, with respect to the Earth. This is a nice geometry for visibility at LOI, especially for a polar orbit, because it lets the LOI burn happen in full view of the ground. With equatorial spacecraft (LADEE) getting the LOI in view of the Earth can require a bit more work. It’s not a great geometry for watching the maneuver, given that there won’t be much radial-rate component, but it’ll do.
Now let’s take a closer look at the Earth to see what is visible.
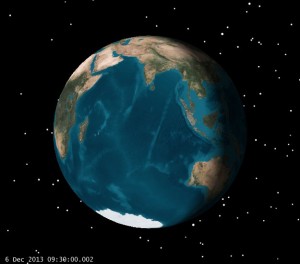
View of Earth from Moon at LOI (click to zoom)
Without digging up the locations of the ground stations, it’s pretty clear that major portions of China are visible, as is Australia. Since we know Chang’e 3 is using some ESA stations, this is set up for a multiple ground stations to see LOI. Nice geometry for Orbit determination and real-time tracking of the event.
After one rev in Lunar orbit, we can see what the orbit looks like here:
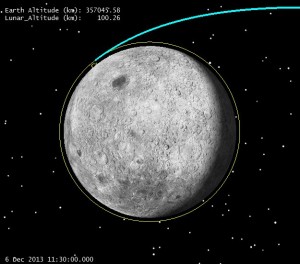
Lunar Orbit 1 Rev past LOI (click to zoom)
The next question is, how does this set us up for landing? We have approximated the landing site at the Sinus Iridum region, with a Lunar Latitude of 43 deg N, and a Lunar Longitude of 31 Deg W. Note the lighting of that site at LOI:
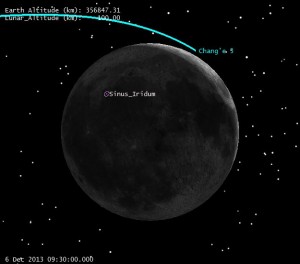
Sinus Iridum landing site lighting at LOI (click to zoom)
Let’s look at the first day’s worth of ground tracks on the Moon, which will help us see what we’re waiting for, both in terms of lighting and geometry:
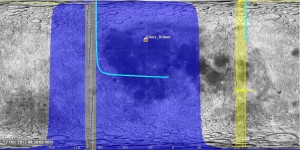
Chang’e 3 Ground track 1 day after LOI (click to zoom)
Note that the light blue line shows the incoming trajectory and the subsequent lines show the progression of ground tracks (which move from right to left). Further note that part of our ground track is in shadow (blue) as is the landing site, and part is in sunlight. Obviously, for landing we’d like sunlight both on the site and on our ground track. Here are the tracks a day later:
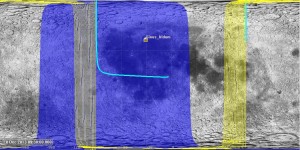
Chang’e 3 Ground track 2 days after LOI (click to zoom)
Our sunlit ground track on the right is moving closer to the landing site, and the shadow is drifting in the right direction as well. The trick here is to just wait in orbit while the Moon rotates under us. Looking at this in 3D gives a better geometrical perspective:
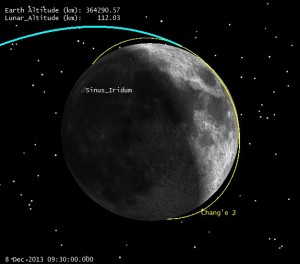
Chang’e 3 landing site and shadow geometry 2 days after LOI (click to zoom)
The orbit is pretty much fixed in inertial space, and we just have to wait while the Moon rotates. If we wait until Dec 15th we get this:
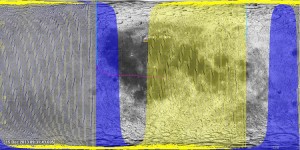
Dec 15 Lunar Ground Tracks (click to zoom)
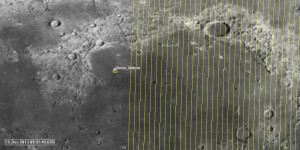
Dec 15 Lunar Ground Tracks Zoomed (click to zoom)
At this point we’ve all but gotten lined up with the landing site, and it’s time to start the descent. Now we have to do a bit of guessing. We know that the descent profile goes from a 100 km circular orbit to a 100 x 15 km orbit (altitudes). We know that the vehicle lowers to periselene and then lowers from there. We’re going to assume that we won’t go a full rev in the 100 x 15 km orbit, but will instead just execute one half rev and descend. If so, it looks like this:
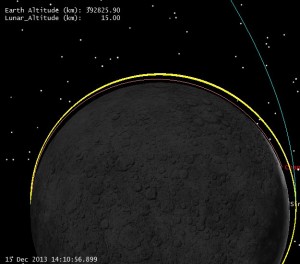
Descent Orbit Initiation to Peri (click to zoom)
It’s hard to show on this scale, but if you zoom and look closely at the left, you can see the orbit starts at 100 km (yellow) and then descends to 15 km on the right. Let’s look from the landing site perspective. Note we can see the approach hyperbola still (blue) the 100 km parking orbit (yellow) and the spacecraft at Periapsis just peeking over the limb at the top. You can see that the parking orbit isn’t completely locked inertially, it’s got a bit of drift in it from the Lunar gravity field.
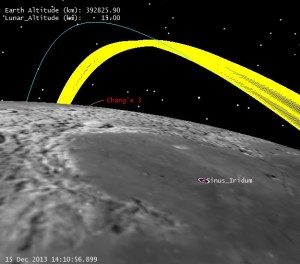
Descent to Periapsis from Landing Site (click to zoom)
Now I have to really fake it. According to the superb site Spaceflight101, the landing engine actively throttles all the way down:
Spaceflight101 Chang’e 3 Flight description
While I can model an engine that throttles, it’s way too much work (if I was working on the mission, I’d have the lander controls people do this) so I’m going to fake it with 2 constant thrust finite burns and a coast segment. I won’t bother trying to get masses and engine masses right either, I just want to show the basic idea:
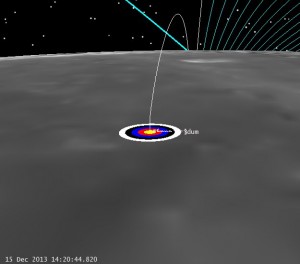
C-3 Landing (click to zoom)
Of course the real profile won’t be exactly like this, but this is a reasonable facsimile. Let’s check out the geometry with respect to the Earth:
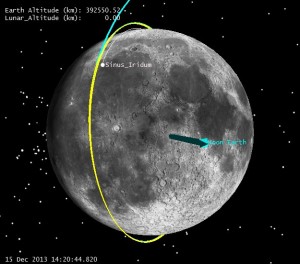
Earth Vector at Landing (click to zoom)
And finally let’s see what’s visible from Earth at the landing time:
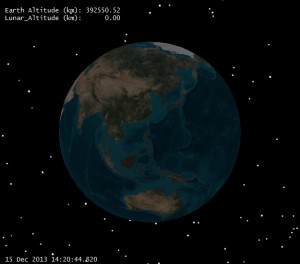
View of Earth from Moon at Landing time
Which looks pretty darn good if you want to get coverage from Chinese ground stations. (Note: I lit the Earth up a bit in this picture to show what was visible, but this half of the Earth is in darkness at the landing.)
Pretty fun stuff. We welcome any updates, if anyone has better data than we do, it’s real easy to change the assumptions and re-run.
-Astrogator_Mike
by John Carrico | Oct 25, 2013 | General Comments
Part of an astrogator’s job is to determine the spacecraft’s current orbit, and predict where it will be in the future, just as the navigators on sailing ships figured out where their ship was and where they were going. Those navigators used star sightings, Sun observations, and accurate clocks to figure out their latitude and longitude. One of the ways they calculated their speed was to drop floats into the water and time how long it took for the ship to move a measured distance. On LADEE we also take observations and measurements to figure out where LADEE is. These measurements are called tracking data, which we receive from many ground tracking stations which are positioned around the world. We use large dish antennae from NASA’s Near Earth Network, NASA’s Deep Space Network, and from the commercial Universal Space Network. We schedule time each day for the various ground stations to track LADEE, using radio signals, and they send us tracking data files containing their measurements during and after the pass. In these files we can get the ground station’s observations of the distance to the spacecraft (“Range”); the speed at which the spacecraft is moving towards or away from the ground station (“Doppler” or “Range Rate”); and the angles in the sky where LADEE is, from the ground stations’ point-of-view. We don’t always get all these types of measurements, nor do we get tracking data all the time, but we don’t always need to. (We will never say no to more tracking data, though. We like it when the controllers schedule extra time to communicate with the spacecraft because then we get more tracking data! We often exclaim, “More Data!”) After we get the tracking data, we use a first guess at what we think the orbit is currently, and we estimate a new orbit by adjusting the trajectory slightly to fit the new measurements. We use AGI’s Optimal Extended Kalman Filter, (in Orbit Determination Tool Kit, aka “ODTK”) to read these files and estimate LADEE’s orbit. We’ve used this on many other cislunar and Earth orbits before, and it really works great!
The end result of our orbit determination work is a spacecraft ephemeris file of where LADEE has been in the past, and another ephemeris file of the predicted orbit, which is where we think LADEE will be in the future. The ephemeris file of the past trajectory is sent to the scientists who need to know where LADEE was when it took their science measurements. The predicted ephemeris file is used by the mission planners for scheduling their activities. It’s also used by other astrogators to calculate “acquisition” data to send to the ground stations so they know where and when to point their antenna to track LADEE. And it’s used by other astrogators to plan the next orbit maneuvers and attitude orientation plans.
It’s very important that we calculate accurate orbits, and we have some pretty tight requirements on how accurate our past orbit knowledge must be, as well as how accurate our predicted orbit must be. As you might imagine, since the tracking is done using radio waves, the signals can have noise on them. The signals are affected by things like weather (one of the ground station dishes was struck by lightning a few weeks ago!). The electronics on the spacecraft also are affected by the harsh temperature changes in space. To make sure our orbit is accurate we spend a lot of time “tuning” the filter settings. This includes identifying and throwing out bad data, calculating how much noise is on each measurement, and calibrating bias values for the various tracking electronics. Also, every time there is a maneuver, whether a large orbit burn, or a small momentum dump, we have to calibrate that maneuver and estimate its exact magnitude and direction. It’s been very rewarding working with the other LADEE astrogators that do this orbit determination work: Craig Nickel, Ryan Lebois, and our Orbit Determination lead, Lisa Policastri. They have done a great job, preparing for years before we launched, and since have been working long hours on crazy shift schedules during operations to make sure we know where we are and where we are going! We have a lot of discussions on how the data look, what the biases might be, and things to try to make our orbit solutions better. (Some conference papers we’ve written describing the details of how we’ve done Orbit Determination for other missions can be found at http://www.applieddefense.com/resources/white-papers/ )
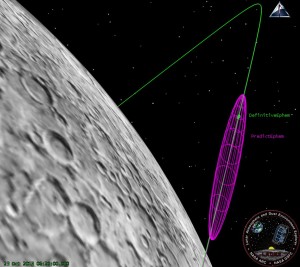
The LADEE OD team compares the orbit prediction (Purple) from a few days ago with the current solution (Green), making sure that the predictions are accurate enough to support communications and LLCD pointing activities.
After we get an orbit solution, we then go through a lot of self-consistency checks to convince ourselves that our orbit is good, and we check our previous predictions with new data to see how well we are doing (as shown in these figures.) It is frustrating, though, that we can’t just look out a window and see where LADEE really is! Sometimes it seems like we are flying a remote control airplane with a blindfold on, maneuvering by only listening to the sound of the engine as it gets closer or farther from us!
Every once in a while, though, we get a good indication that things are going well. For example, when we were getting ready for our first Lunar Orbit Insertion burn, we were sure everything was going well, but there’s still a lot of anticipation in the air…. we were leaving the Earth’s dominating gravity, and falling rapidly towards the Moon. (“Falling with Style” as Buzz Lightyear would say!) We designed LADEE’s trajectory to go behind the Moon and—based on our predictions—we planned the exact time for the main engine to fire to brake into Lunar orbit, igniting just a few minutes after LADEE became visible again from behind the other side of Moon. We sure hoped we got the prediction correct! In addition to estimating the time LADEE would lose signal as it disappeared behind the Moon, we also estimated the uncertainty in that time; we predicted we knew the time within 2 seconds. We were listening to the other team members on the voice loop, and we cheered as they called out that the Loss Of Signal was within 2 seconds of our prediction!
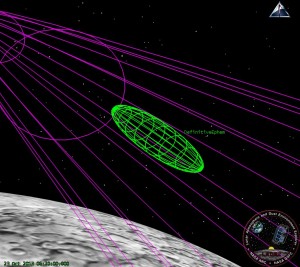
The LADEE OD team calculates the uncertainty of each orbit solution. One consistency check we perform is to see that the difference between the predicted and final orbit estimates are close compared to their uncertainties. The uncertainty of the predicted orbit is shown in Purple, and the uncertainty of the current “definitive” solution is in Green. In this case, the final definitive orbit and it’s uncertainty is completely within the predicted uncertainty. This is an indication that the prediction a few days ago is still good to use now.
Then came the Lunar Laser Communication Demonstration (LLCD experiment), a great deep space technology that has now been successfully demonstrated. (A cool video here: http://youtu.be/ptfLfrWI648 and the NASA web page is here: http://llcd.gsfc.nasa.gov ?). When they started the first experiment, it just worked! As quoted in the article from Popular Mechanics: (http://www.popularmechanics.com/science/space/nasa/lunar-laser-communication-experiment-succeeds-16068586):
“We thought the ground terminal would have to do a little searching, but it turned out it was pointed perfectly,” Boroson says. “We turned it on and all cheered.” The connection was almost instantaneous. After the spacecraft and ground terminal connected, a 4-inch laser beam travelled 238,000 miles from the moon to New Mexico.
And in the article from Spaceflight Now: (http://spaceflightnow.com/minotaur/ladee/131023laser/#.Ummt-_mTh8F):
Over the 239,000-mile distance between the Earth and the moon, the 4-inch-diameter laser column disperses to a width of 3.5 miles by the time it reaches the ground.
There was really little room for error: the pointing had to be correct, and the predicted ephemeris has to be accurate. Although we had done several tests prior to launch, sending test products to check out the system, we didn’t know how things would work until they turned on the LLCD system. You can imagine when we heard over the voice loops and in the status briefings last weekend that the orbit ephemeris was good enough that the system worked right away, and that they didn’t need to search, we were very excited!
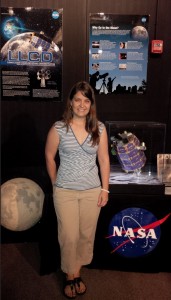
Our LADEE Orbit Determination lead, Lisa Policastri, visited the NASA Wallops Flight Facility prior to launch. She is standing here in front of the LADEE display, and you can see the LLCD display on the left.
The orbit determination team really did their job! And, in addition, our nine-person Flight Dynamics Team performed several other tasks to make the LLCD experiment work. We calculate the orientation (also called the “attitude”) of the spacecraft for the experiments. We also create the on-board spacecraft ephemeris so the LLCD instruments mounted on LADEE know where and when to point back at the ground terminals on Earth. And we generate the pointing angles for the ground terminals to point their LASERS at LADEE. Of course, we’re not the only ones… Our flight dynamics team sits in the room next to the activity planners, and the many sub-system engineers, and we are near the controllers, and the many other folks that worked through the weekend and over long nights at Ames to make this successful. It was an exciting weekend!
And that’s just listing some of the folks at NASA Ames. To see the rest of the LLCD team, check out the web page http://llcd.gsfc.nasa.gov – It really is great to see what can be accomplished when so many people, of very different talents, have a chance to work together, and try something new.
by Astrogator Mike | Oct 22, 2013 | General Comments
Sorry for the delay in updating, Astrogator_Mike had to make the long drive home after LOI-3 and get configured back in his home base. In the mean time, Lunar Orbit Insertion Burn 3 (LOI-3) was completed successfully (within 0.6% of target) on Oct. 13, 2013. LADEE’s post LOI-3 orbit had an aposelene altitude of 250 km, and a periselene altitude of 235 km. You can see below that this has evolved over time to 220 km x 260 km.
LADEE will now stay parked (i.e. no maneuvers) for roughly a month, while the spacecraft does alternating Lunar Laser Communication Demonstration (LLCD) tests, and calibration tests of the other science instruments.
In fact, the LLCD has been doing really well, breaking records and having all sorts of fun: NASA Is Now Communicating With the LADEE Spacecraft Via Laser
LADEE’s current view looks like this:
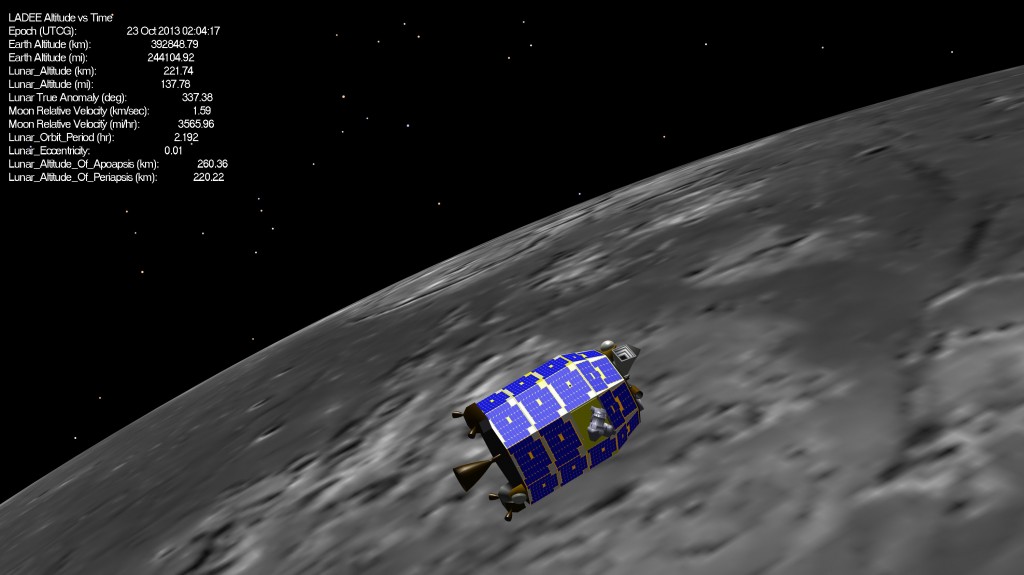
Which looks like this from above the orbit:
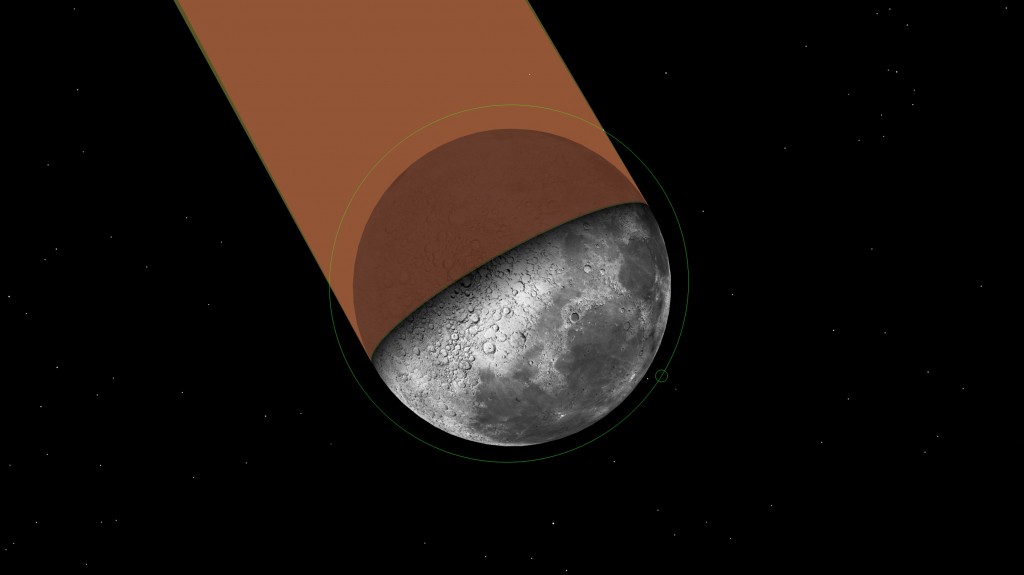
And from the Earth looks like this:
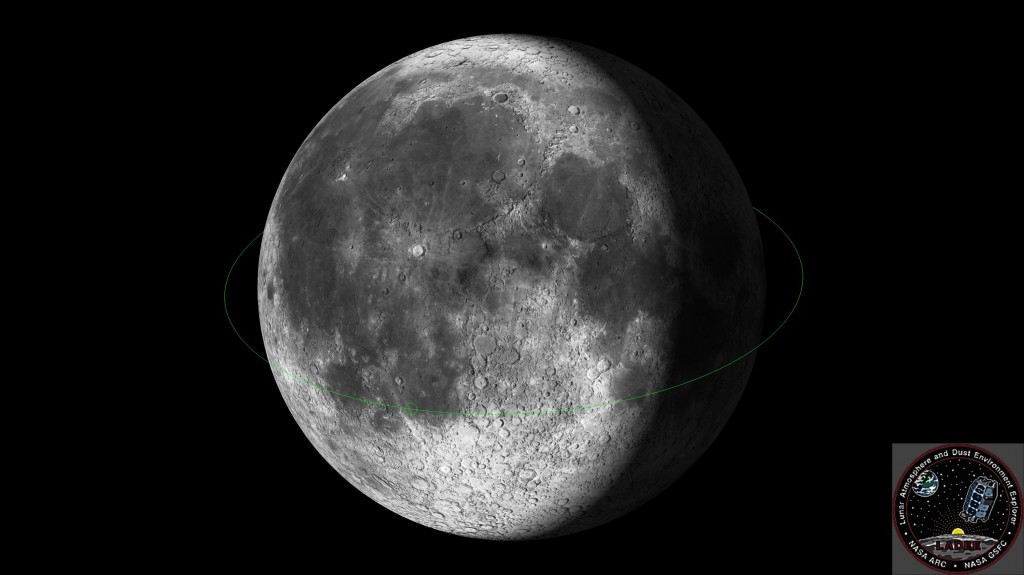
Note that you can zoom in on any of these pictures by clicking on them.
by Astrogator Mike | Oct 9, 2013 | General Comments
The LADEE Lunar Orbit Insertion burn 2 (LOI-2) executed as planned this morning at 3:38 PDT, placing LADEE into a 4 hr orbit. Things move much faster now for the spacecraft, and the Moon is looking a lot bigger. We originally captured with a periselene altitude near 560 km but our periselene has now been lowered to an altitude of ~235 km by Earth perturbations in the Post-LOI-1 24 hr orbit. The planned periselene of the commissioning orbit was 250 km, however the small (<1%) underperformance of LOI-1 caused aposelene to be slightly higher, and thus we got slightly more Earth perturbations than we nominally planned for. The result of this is that we got a bit of free lowering from the Earth, which we’ll take! (Since we plan to go lower than 250 km anyway). So the current plan is to drop the aposelene to 250 and perform commissioning there in the 235 x 250 km orbit.
LOI-2 lowered our apogee down to ~2200 (we’ll have to wait for some more tracking to verify that exactly).
From our pre-LOI2 planning, things should now (9 Oct 2013 13:00 UTC) look like this:
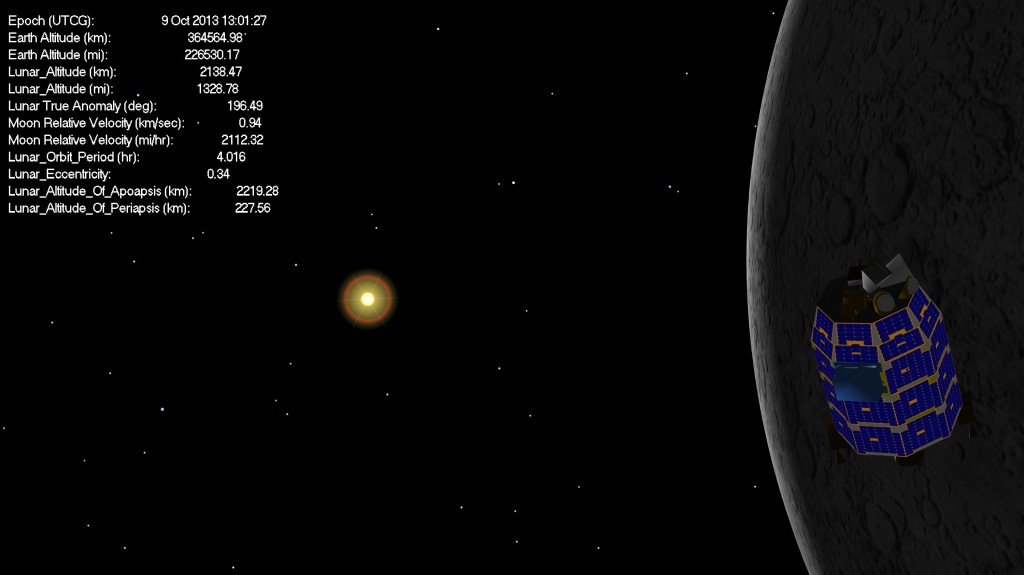
And we are here in the orbit:
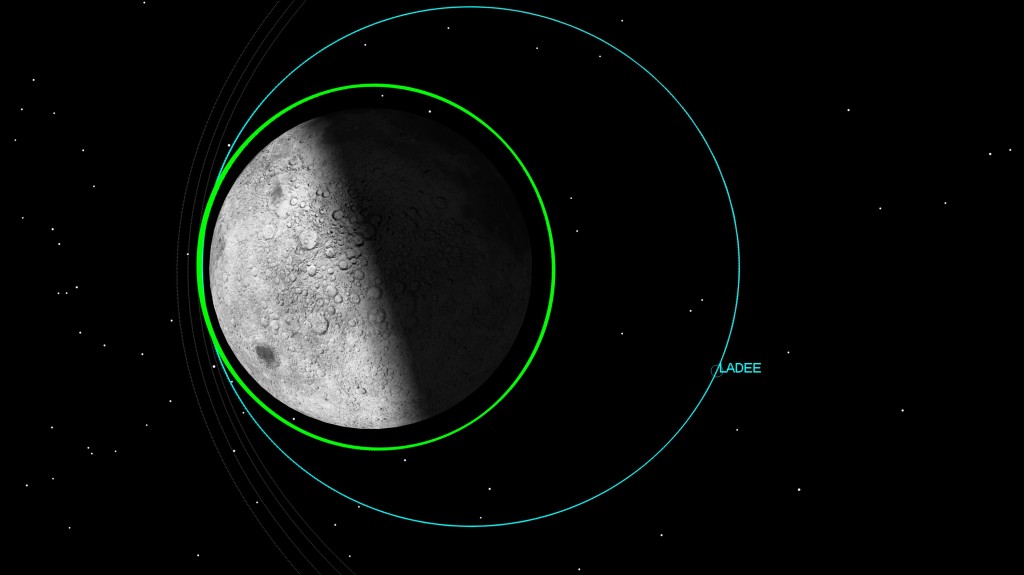
If you could see the orbit from Earth you’d see this:
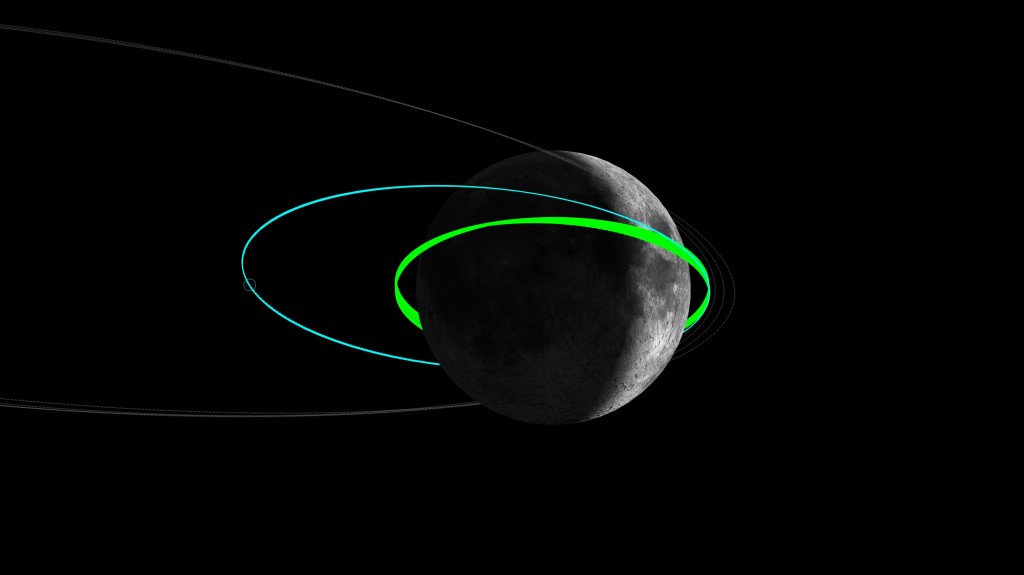